
Sustainable Agriculture Student Research Project

Potential of halophyte phytoextraction for saline soil remediationSarah-Jane Copeland, Department of Sustainable Agriculture and Food Systems, 2020 |
Introduction
Viable agricultural land is increasingly being lost to salinity as a result of natural and anthropogenic causes. It is estimated that 7% of total land area around the globe is affected by salts, and that 12 million ha of irrigated agricultural land may become futile as a result of high salt content. Soils with a high concentration of sodium, calcium, and potassium ions experience adverse effects on soil structure and fertility, which greatly impacts plant health and productivity. The use of halophytes as a mode of phytoremediation may be an ecologically sound and cost-effective alternative to traditional engineering-based remediation methods.
Phytoremediation: a form of green remediation that utilizes living plants to harness and debilitate pollutants surround the plant rhizosphere.
Phytoextraction: a class of phytoremediation where soil pollutants are absorbed by plant roots and sequestered into their plant tissue.
Halophyte: a group of extremophiles that thrive in saline / sodic conditions unsuitable to most plants. General features:
- Quick growing
- Produce significant biomass
- Long root systems
- Succulent vegetative structures
- Drought tolerant
Objectives
This study aimed to explore the potential of phytoextraction as a means of saline soil remediation by the halophyte Salsola komarovii, in comparison to the salt-sensitive glycophyte Phaseolus vulgaris. The key points of the study were to:
- Compare the difference over time in soil electroconductivity between a halophyte (S. komarovii) and glycophyte (P. vulgaris).
- Study variation in plant growth with an increasing ratio of salts.
- Explore the relationship between increasing salinity and biomass in order to determine if halophyte development is correlated to salt content.
Methods
Location: KPU Farm, Garden City Lands, Richmond, BC.
Experimental design: Randomized complete block split-plot design.
- Four replicate blocks (2 x 8 m), divided into 16 main plots (2 x 2 m) and 64 sub-plots (1 x 1 m)
- Four main treatments:
- Control: no added spent mushroom compost mulch
- 5 cm spent mushroom compost mulch
- 10 cm spent mushroom compost mulch
- 20 cm spent mushroom compost mulch
Spent mushroom compost (SMC) was obtained from Highline Mushrooms. Its high salinity made it a source of soluble salts. The compost is considered spent as it is the residual waste left after the spawning, casing, and harvesting phases of mushroom production.
- Seedlings of P. vulgaris and S. komarovii were started indoors and field transplanted June 19th, 2020. Each sub-plot received two transplants of the same species.
- Data were collected bi-weekly between transplanting and August 28th, 2020.
Data collection:
- Electroconductivity: Soil electroconductivity (EC) was measured using an EC meter (mS/cm).
- Biomass: Marketable plant fresh weight (kg) was measured upon completion of the experiment by harvesting mature pods of P. vulgaris and cutting S. komarovii at the soil level.
Statistical analysis: All data were analyzed using jamovi statistical software
- Electroconductivity: Data were analyzed using a linear mixed-model approach. Fixed effects were crop type and depth of SMC; random effects were main plot and replicate.
- Biomass: Data were analyzed by regression analysis. The independent variables were replicate, crop type and depth of SMC, while the dependent variable was plant fresh weight (kg).
Image
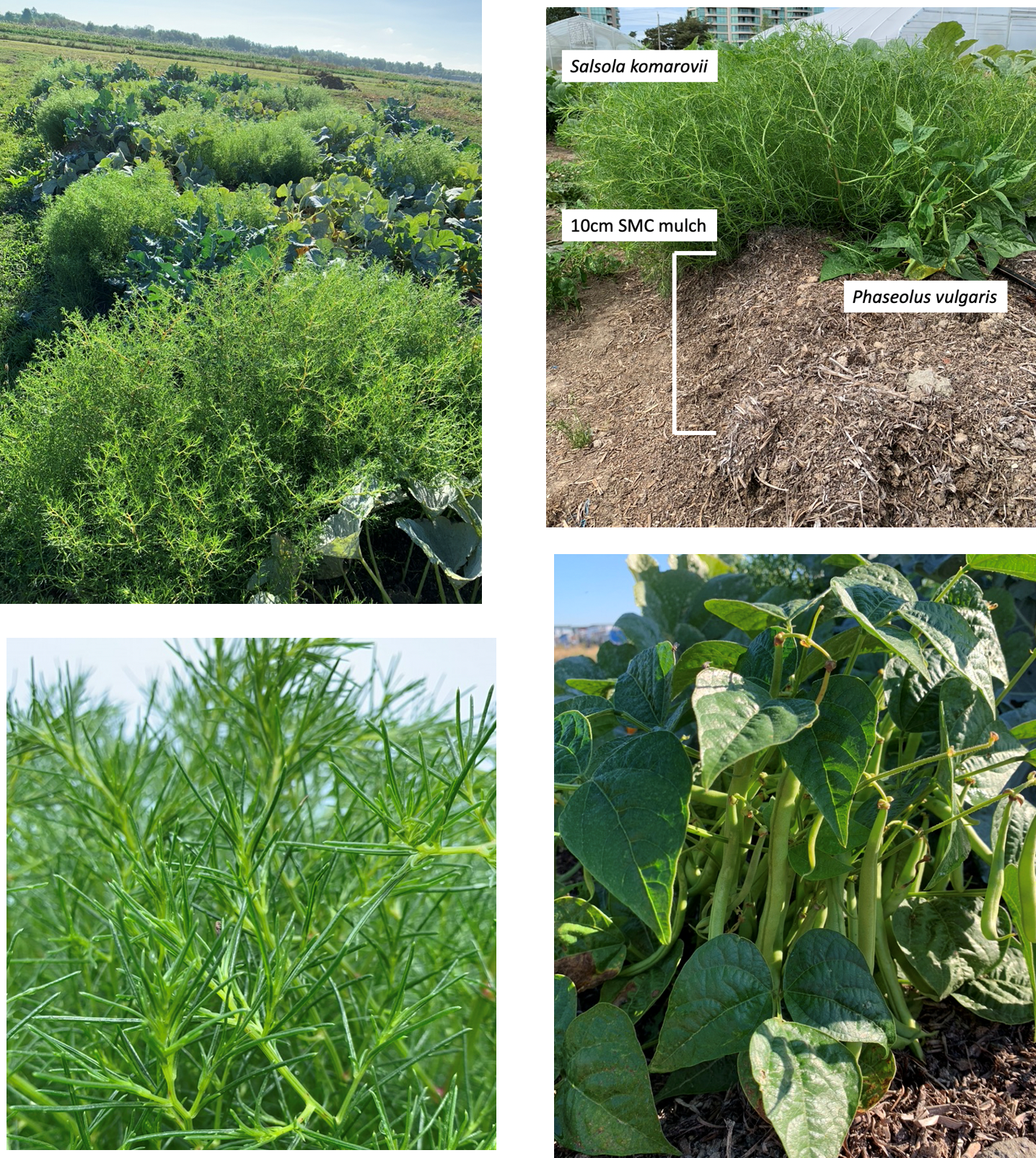
Results
- Electroconductivity: Soil EC was significantly (p ≤ .05) higher in the SMC treated plots compared to the control plots (Fig.1). Soil EC increased with SMC depth for both P. vulgaris and S. komarovii. No significant two or three-way interactions were observed between crop, mulch depth, and observation date (Fig.2).
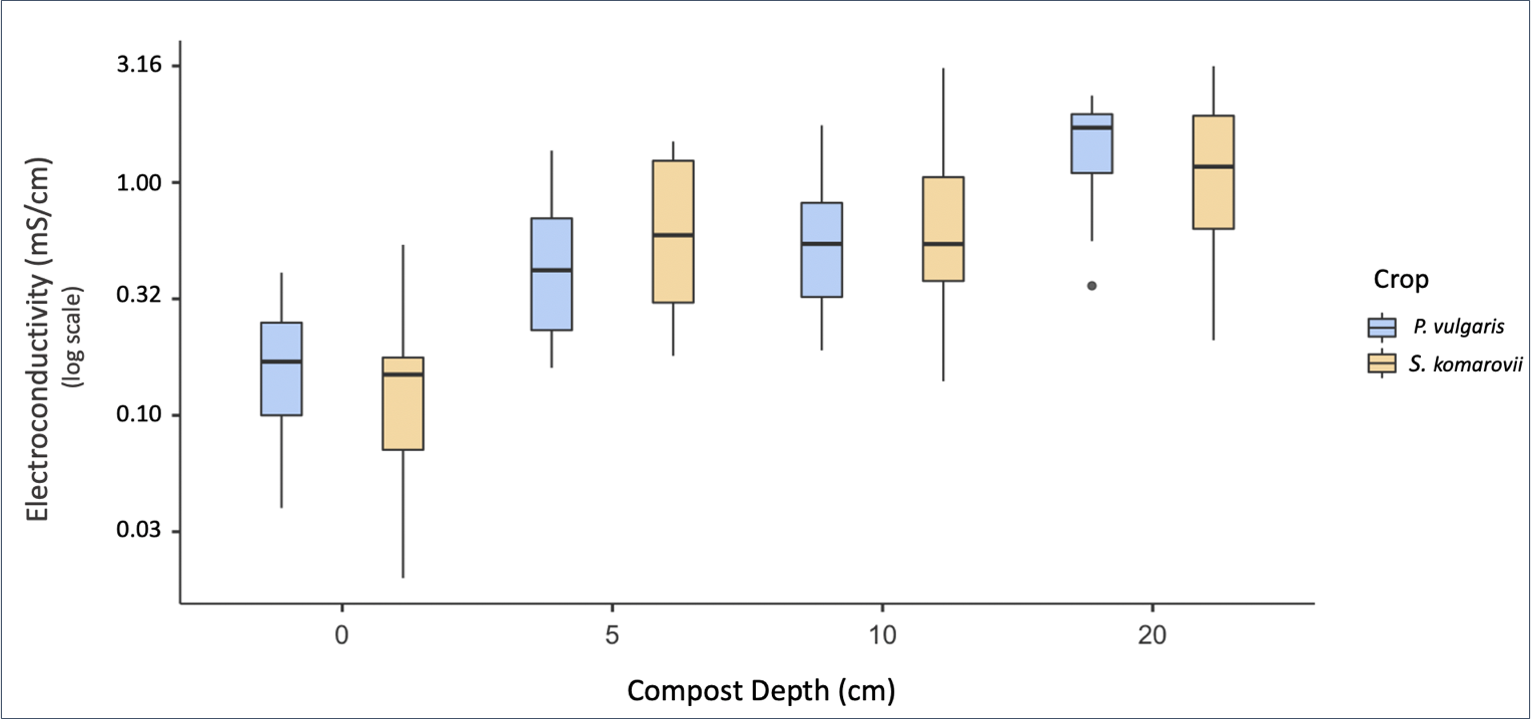
Figure 1. Effects of increasing compost depth on soil electroconductivity
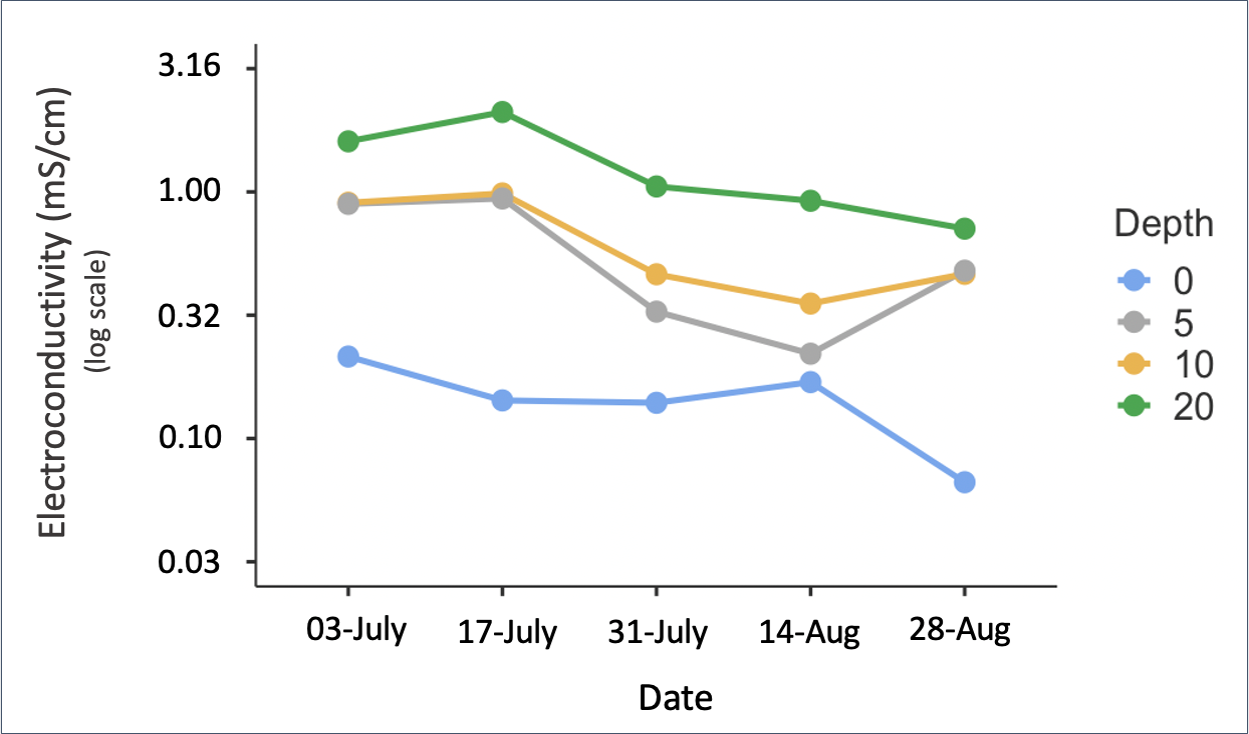
Figure 2. Change in soil electroconductivity over 10 weeks of treatment
Biomass: Plant fresh weight was significantly (p ≤ .001) higher in the SMC treated plots compared to the control plots for both P. vulgaris and S. komarovii. The only significant interaction was between crop and mulch depth at the 0–5 cm level (Fig.3).
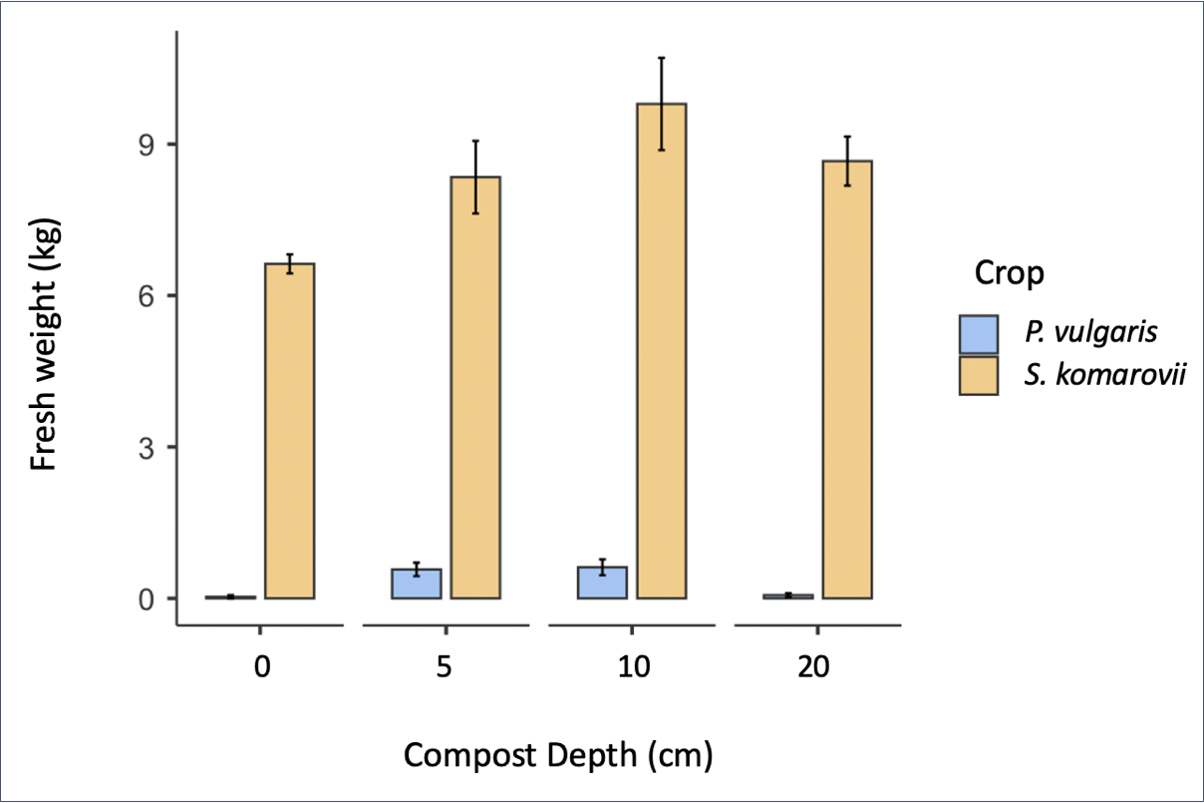
Figure 3. Fresh weight by mulch depth and crop ten weeks after transplanting.
Conclusion
Despite the difference in salt tolerance mechanisms between halophytic and glycophytic species, the results of the experiment do not support the two hypotheses that:
- Soil electroconductivity would change depending on crop type (halophyte vs. glycophyte) over the course of the trial.
- Plant growth of P. vulgaris would decline at a greater rate than S. komarovii with increasing salinity.
Utilizing halophytes as a form of phytoremediation is still in the preliminary stage and future research will continue to reveal its feasibility under various circumstances.
Acknowledgments
I would like to thank my professor Mike Bomford for his extensive knowledge and guidance throughout the duration of this experiment. Notably, for his extra input as a result of the Covid-19 pandemic by starting and maintaining the plant seedlings and establishing the research plots. I would also like to thank my classmates Maria Santander Mercado and James Oswald for their collective help in maintaining the research plots, collecting data, and harvesting the final plants. Thanks to Highline Mushrooms for providing the spent mushroom compost used in this study.
Funding and Support:
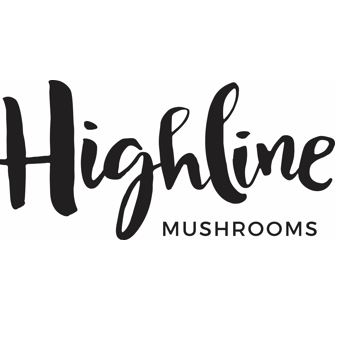